It is possible to image an object with an induced coherence effect by making use of photon pairs to gain information on the item of interest—without detecting the light probing it. While one photon illuminates the object, its partner alone is detected, thereby preventing the measurements of coincidence events to reveal information of the sought after object. This method can be made resilient to noise, as well.
In a new report published in Science Advances, Jorge Fuenzalida and a team in applied optics, precision engineering and theory communications in Germany experimentally showed how the method can be made resilient to noise. They introduced an imaging-distilled approach based on the interferometric modulation of the signal of interest to generate a high-quality image of an object regardless of the extreme noise levels surpassing the actual signal of interest.
Quantum imaging
Quantum imaging is a promising field that is emerging with valid advantages when compared to classical protocols. Researchers have demonstrated this method across different scenarios to work in the low-photon flux regime by making use of undetected probing photons for super-resolution imaging.
Scientists can also develop protocols in quantum imaging without a classical counterpart based on quantum interference and entanglement. Quantum imaging protocols can, however, be made resilient to noise. For instance, distillation or purification can remove decoherence introduced by the environment in a quantum system.
It is also possible to implement quantum imaging distillation with one and several photon pair degrees of freedom. In this work, Fuenzalida and team introduced and experimentally verified a quantum imaging distillation method to detect single photons only.
The method of quantum imaging with undetected light (abbreviated as QIUL) offers a two-photon wide-field interferometric imaging method. During this process, one photon can illuminate an object, while only its partner photon is detected on the camera. Incidentally, the photon illuminating the object remains undetected.
The method offers a unique discovery method to probe samples. The scientists then introduced a source of noise in the quantum imaging scheme to study its resilience to show good performance even for noise intensities above 250 times the quantum signal intensity.
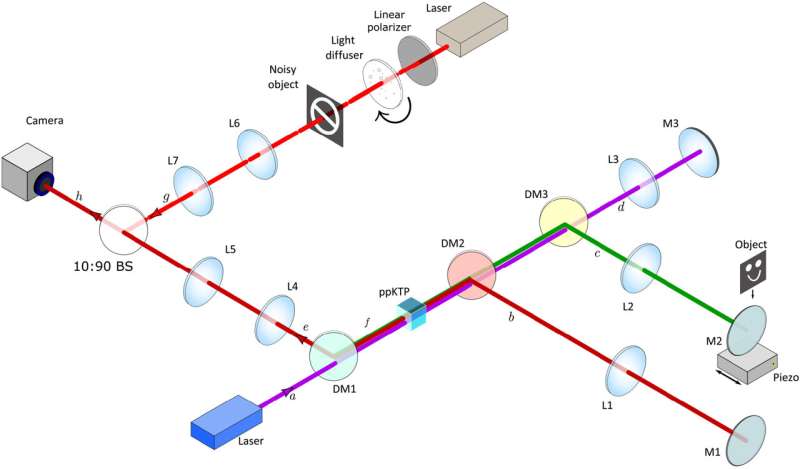
Cleaning a quantum image and generating a photon pair
Quantum imaging distillation is a method in use to clean a quantum image from noise. The team illustrated the distillation method while defining a noise image as an unwanted signal superimposed over a quantum image on the camera. To distill an image, Fuenzalida and team used quantum holography with undetected light (abbreviated QHUL), where the object information was carried into a single-photon interference pattern. If the intensity difference of the method is bigger than the intensity variance of noise, the team could distill the quantum image.
To generate photon pairs mediated by the interaction of an intense pump beam with the atoms of a nonlinear crystal, the team used spontaneous parametric down-conversion. The imaging scheme used an interferometer to generate a pair of signal-idler photons in the forward and backward propagation modes. The noise variance in the setup contributed to the signal intensity variance; where a difference of signal intensity higher than the noise variance can distill the quantum image.
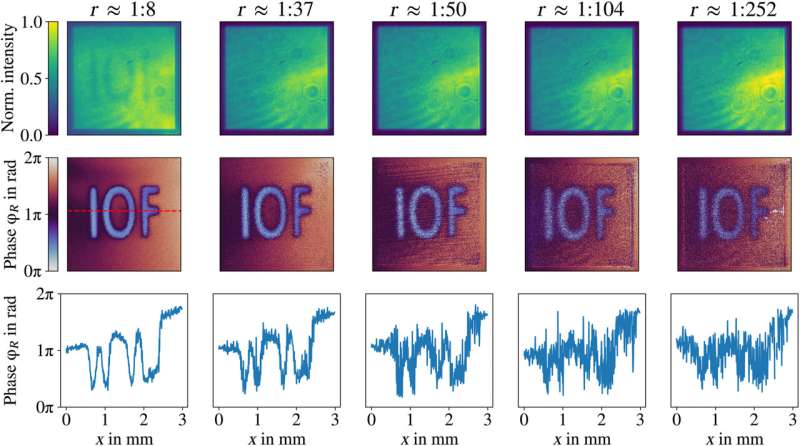
Experimental nonlinearity and noise sources
Fuenzalida and colleagues implemented an experimental setup using a nonlinear interferometer in a Michelson configuration and pumped a crystal with a continuous wave laser. Due to the strong nonlinearity of the experiment, the team generated a photon pair via spontaneous parametric down-conversion along the paths, although never simultaneously. They separated the signal, idler, and pump beams in the forward propagation direction by using dichroic mirrors and reflected into the crystal with a series of mirrors.
The camera in the experimental framework showed an interference pattern of signal photons, which the team noted as the transfer of object information obtained by the idler photon to the signal photon interference pattern. The team used a continuous wave diode laser with a variable pump power to introduce noise into the system, and varied the properties of classical illumination, intensity and variance to examine the effects of noise and the distillation performance.
Distillation performance across diverse noise intensities
The scientists superimposed classical and quantum images to perform quantum holography with undetected light to distill or clean the quantum image under diverse intensities of noise. For the quantum image, they used signal photons generated in a single pass through a crystal, where the signal intensity did not change during the experiments. They characterized different noise intensities by superimposing quantum and classical images on the camera, and as the noise intensity increased, they measured the accuracy of the experimental results.
The researchers conducted a second experiment to quantify the effects of varying noise on the phase accuracy of distilled images by using similar configurations of noise intensities. The experimental behavior was in good agreement to theory, and compared well to existing methods.
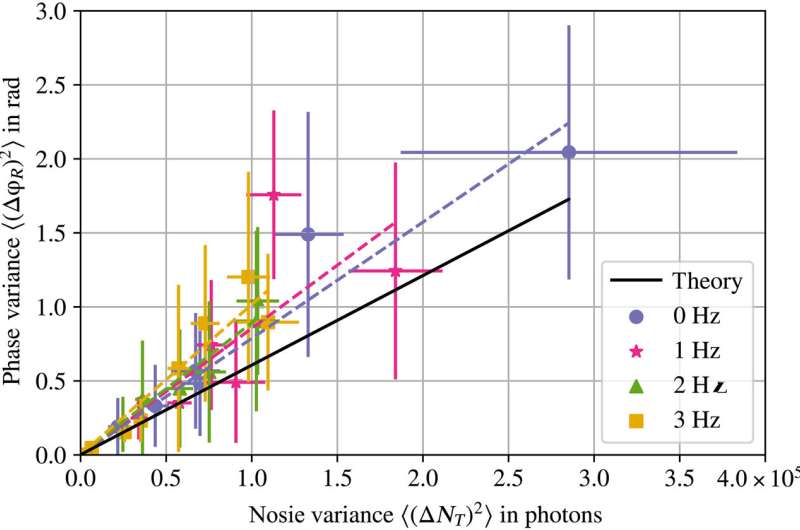
Outlook
In this way, Jorge Fuenzalida, and colleagues investigated quantum imaging with undetected light (QIUL) in a two-photon wide-field interferometric imaging method. While one photon illuminated the object of interest and its partner remained on the camera, the illuminating photon remained undetected. The scientists distilled or cleaned the image by using quantum holography with undetected light (QHUL). They proved the imaging method by superimposing partially or completely a classical source of noise on top of the quantum image on the camera. The method worked every time, even with noise intensities higher than the signal intensity.
The team explored the limits of the method by presenting simulations of quantum holography under extreme noise scenarios. The experimental outcomes provide a step forward for quantum imaging in open systems to even examine the limits of innovative versions of quantum-based light detection and ranging (LIDAR), by using undetected light.
#QuantumLight #QuantumOptics #QuantumFuture #QuantumInnovation
Visit:https://quantumtech.sfconferences.com/
Award Nomination:https://x-i.me/rmRF
Instagram:https://www.instagram.com/sahara_navi/
Pinterest:https:https://in.pinterest.com/quantumtechnology23/
Blogger:https://quantumtech23.blogspot.com/
Facebook:https://www.facebook.com/profile.php?id=100090774864954
Tumblr:https://www.tumblr.com/quantum-technology